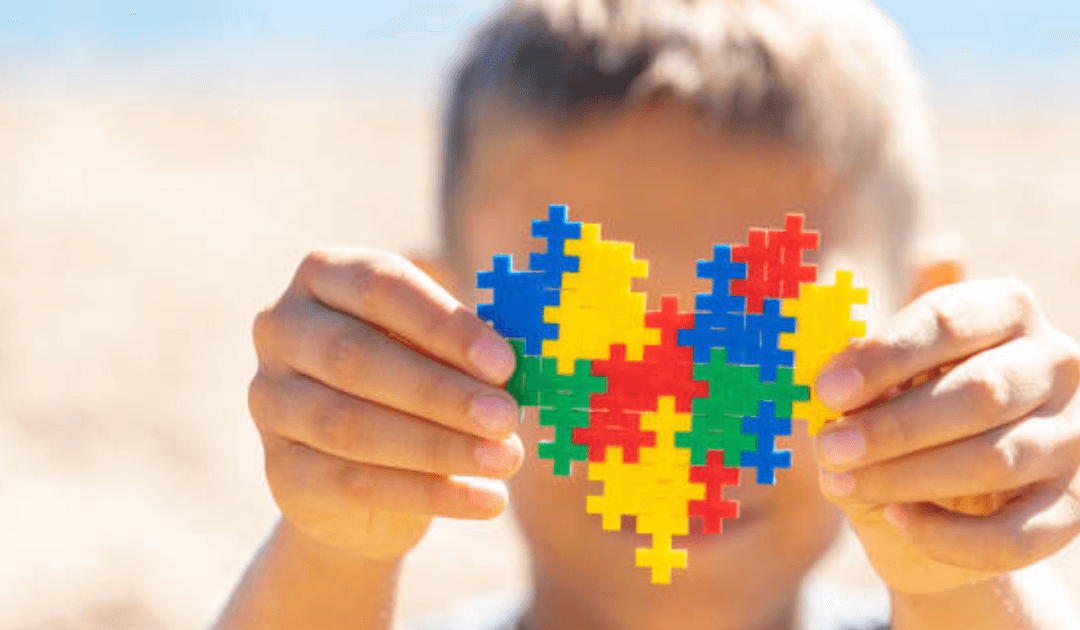
General Symptoms of Autism
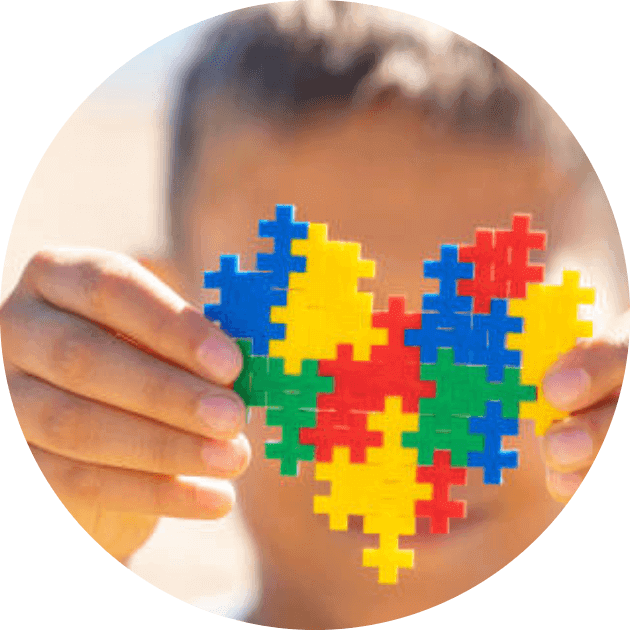
Autism, also known as autism spectrum disorder (ASD), is a developmental disorder that affects communication and social interaction. The severity of ASD can vary widely, and different individuals with ASD may experience different symptoms.
What are the classic symptoms of autism?
- Difficulties with social interaction: Children with ASD may have difficulty engaging in social interactions, such as making eye contact, initiating or maintaining conversations, or understanding social cues.
- Repetitive behaviors or interests: Children with ASD may have repetitive behaviors or interests, such as lining up toys or objects, or exhibiting strong interests in a specific topic.
- Communication challenges: Children with ASD may have difficulty with communication, including delays in language development or difficulty using language to communicate their needs or desires.
- Sensory processing issues: Children with ASD may have sensory processing issues, such as oversensitivity to certain sounds or textures, or under-sensitivity to pain or temperature.
- Unusual responses to sensory stimuli: Children with ASD may have unusual responses to sensory stimuli, such as a fascination with lights or a strong aversion to certain sounds or textures.
What are the symptoms of autism in older children
The symptoms of autism in older children are generally similar to those seen in younger children, although the specific symptoms and their severity can vary widely from one individual to another. Some common symptoms of autism in older children may include:
- Difficulty with social interactions: Older children with autism may have difficulty engaging in social interactions, such as making eye contact, initiating or maintaining conversations, or understanding social cues.
- Repetitive behaviors or interests: Older children with autism may engage in repetitive behaviors or have strong, narrow interests.
- Communication challenges: Older children with autism may have difficulty with communication, including delays in language development or difficulty using language to express their needs or desires.
- Sensory processing issues: Older children with autism may have sensory processing issues, such as oversensitivity to certain sounds or textures, or under-sensitivity to pain or temperature.
- Unusual responses to sensory stimuli: Older children with autism may have unusual responses to sensory stimuli, such as a fascination with lights or a strong aversion to certain sounds or textures.
Here you can find some of the early symptoms
- Lack of eye contact: Children with autism may avoid making eye contact or may not respond when someone else is looking at them.
- Delays in language development: Children with autism may have delays in language development or may have difficulty using language to communicate their needs and desires.
- Difficulty engaging in social interactions: Children with autism may have difficulty initiating or maintaining social interactions, such as playing with other children or engaging in back-and-forth conversations.
- Repetitive behaviors: Children with autism may engage in repetitive behaviors, such as hand-flapping or repeating words or phrases.
- Unusual responses to sensory stimuli: Children with autism may have unusual responses to sensory stimuli, such as a fascination with lights or a strong aversion to certain sounds or textures
Resources
- m B-R, SeoH-S, Ku J-M, et al. Silibinininhibits the production of pro-inflammatory cytokines through inhibition of NF-κB signaling pathway in HMC-1 human mast-cells. Inflammation. Research. 2013;62(11):941-950. doi:10.1007/s00011-013-0640-1.
- ChapowalA. PetasitesStudy Group. Randomisedcontrolled trial of butterbur and cetirizine for treating seasonal allergic rhinitis. BMJ 2002;324:144-6.
- Hayes, N. A. and Foreman, J. C. The activity of compounds extracted from feverfew on histamine release from rat mast-cells. J Pharm Pharmacol1987;39(6):466-470
- Hsieh et al. Baicalein inhibits IL-1ß- and TNF-a-induced inflammatory cytokine production from human mast-cells via regulation of the NF-?B pathway. ClinMolAllergy. 5: 5. 2007.
- TheoharidesTC, Patra P, Boucher W, et al. Chondroitin sulphateinhibits connective tissue mast-cells. British Journal of Pharmacology. 2000;131(6):1039-1049. doi:10.1038/ sj.bjp.0703672.
- Ro JY, Lee BC, Kim JY, et al. Inhibitory mechanism of aloe single component (alprogen) on mediator release in guinea pig lung mast-cells activated with specific antigenantibodyreactions. J PharmacolExpTher. 2000;292:114–121. 73.
- https://www.ncbi.nlm.nih.gov/pubmed/24477254
- https://www.ncbi.nlm.nih.gov/pubmed/28458279
- https://www.ncbi.nlm.nih.gov/pubmed/9421440
- https://www.ncbi.nlm.nih.gov/pubmed/10344773
- https://www.ncbi.nlm.nih.gov/pmc/articles/PMC4315779/